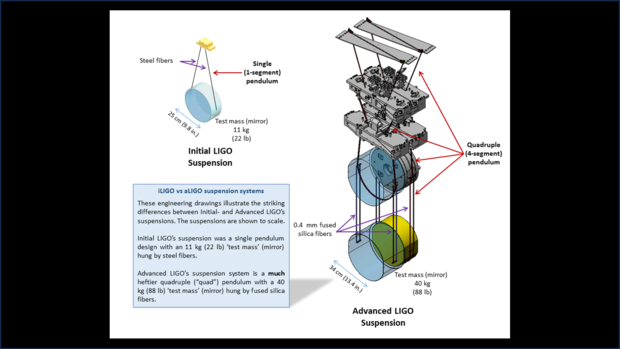
Initial LIGO (iLIGO) test mass suspension compared to the Advanced LIGO (aLIGO) suspension.
Our Evolving Detectors
The history of the U.S. National Science Foundation Laser Interferometer Gravitational-wave Observatory (NSF LIGO) has spanned decades from the moment the LIGO project was approved by the National Science Board in 1990, through selection and construction of the sites and initial instruments, to today, where instrument upgrades continue to be added and implemented between extended observing runs. Given the extraordinary sensitivity and complexity of our detectors, limited, deliberate changes to the instruments must be carefully planned and made to ensure they function at their best so significant scientific gains can be made.
Construction of NSF LIGO's original gravitational wave detectors began in 1994 and 1995 (at the Washington State (WA) site and Louisiana (LA) site, respectively). Dubbed Initial LIGO or iLIGO, construction was completed in 1999. The first search for gravitational waves began in 2002 and concluded in 2010. Initial LIGO's "range" (the radius out to which LIGO could detect at least a binary neutron star (BNS) merger) was 15 megaparsecs (Mpc). A parsec is a unit of distance used by astronomers that equates to 3.26 light years, so a megaparsec is 3.26 million light years, and 15Mpc = 48.9 million light years. LIGO did not detect any gravitational waves during this initial run, but much was learned from the experience to prepare for the next phase of our search for gravitational waves.
Lessons learned during Initial LIGO's operation led to a massive redesign of LIGO's instruments and a new name for the detectors: Advanced LIGO, or aLIGO. Construction of aLIGO components began in 2008, two years before iLIGO was retired. In 2010, Initial LIGO's components were removed and the installation of aLIGO's parts began. The most notable and significant change was made to the test-mass mirror suspensions. The figure below compares Initial LIGO's and Advanced LIGO's "end test mass" suspensions. It took nearly five years to install all of aLIGO's components.
A "test mass" is a mirror that reflects the laser that travels through LIGO's arms and tells us when a gravitational wave has passed. The term "test mass" comes from relativity theory, where it is used to describe a mass free from forces and able to follow distortions of space-time. Since gravitational wave vibrations are so small, the only way to know that test-mass movements are caused by a gravitational wave is to protect them from terrestrial vibrations, even the smallest of which are thousands of times larger than gravitational waves. To do this, we hang the mirrors in pendulum structures equipped with multiple levels of vibration damping systems, and nest these within larger vibration isolation superstructures. Initial LIGO's suspensions were single link pendulums that did not shield the mirrors from a lot of Earthly vibrations. Advanced LIGO's main suspensions have four segments. The qudruple pendulum structure alone reduces external vibrations 100-million times between the top and the bottom. To learn more about LIGO's suspension systems, visit LIGO Technology. |
After the major overhaul transforming iLIGO into aLIGO, LIGO’s search for gravitational waves resumed in September 2015. This time, within days, the aLIGO detectors achieved what Initial LIGO could not accomplish in 8 years of operation: On September 14, 2015, LIGO's interferometers in Livingston, LA and Hanford, WA made the world's first direct detection of gravitational waves generated by two black holes colliding nearly 1.3 BILLION light years away! The first observing run (O1) of this "Advanced Detector Era" lasted just 4 months, and in that time, LIGO successfully detected 3 gravitational waves, all from colliding black holes. More importantly, by the end of O1, LIGO had achieved a BNS range of 80 Mpc, more than five times farther than Initial LIGO.
After each observing run, LIGO's instruments undergo continued improvements and refinements before resuming their search for gravitational waves. Multiple changes are made to the instruments in these intervening periods (sometimes smaller adjustments are made within observing runs, too), each one designed to make the detectors more stable or quiet and generally more sensitive to smaller gravitational waves (those from less energetic sources, or those from much farther away).
Between January and November 2016, aLIGO underwent further improvements to prepare for its second observing run, O2, which began in November 2016 and ended at the end of August 2017. LIGO confirmed 8 detections in this run, including the revolutionary discovery of the first ever detection of two neutron stars colliding. O2's range topped out at just under 100Mpc (a 22% improvement over O1).
After still more extensive instrument upgrades, LIGO's third observing run (O3) began in April 2019, and ended in March 2020. Our instrument range again beat that in O2 by a wide margin, reaching 135 Mpc, nine times farther than what we achieved with Initial LIGO! With this improved performance in sensitivity and in range, in this extraordinary run, LIGO nabbed a whopping 79 detections, demonstrating the value of our continued and careful stepwise upgrades.
By the end of our first three observing runs in the "Advanced LIGO Era", over just 25 months of total observing time, LIGO's ever-improving instruments had detected 90 merger events and improved their range from 80Mpc to 135Mpc!
But LIGO engineers aren't finished improving the instruments. From the beginning of the Advanced LIGO era, one goal has been to improve the range of the interferometers by 10 times over Initial LIGO's 15Mpc. Increasing the range 10 times would increase the volume of space we can probe by 1000 times (volume increases with the cube of the distance, so 10 times farther away equates to 1000 times the volume of space included in the search). LIGO finally achieved this milestone in 2023 during the fourth observing run, O4. O4 began in May, 2023, and both the Livingston and Hanford detectors quickly surpassed the 150Mpc range mark.
Animation showing LIGO's range evolution from Initial LIGO to O4. The map, centered on the Milky Way, shows galaxy clusters within ~150Mpc (500 million light years) radius. In O4, LIGO's reach encompassed all of these clusters. Click the 'full screen' button--lower right corner of video frame--for full-sized graphic. (Map base: Richard Powell, Atlas of the Universe. Animation: Caltech/MIT/LIGO Lab/K. Burtnyk) |
|
The Future of Gravitational Wave Detectors
LIGO's evolution will continue as long as it is in operation, and significant redesigns are already in planning stages, for example, replacing the current 40kg test mass mirrors with 100kg mirrors and much larger suspension systems. Around the world, plans are already being made for the next generation of gravitational wave detectors. The next major step in ground-based gravitational wave instruments will involve much larger facilities. These so-called "Third Generation" or 3G gravitational wave detectors are already being explored, including Cosmic Explorer, an interferometer with 40-km long arms, or Einstein Telescope, a triangular detector with 10km long arms buried deep underground.
Not to be outdone, an enormous space-based gravitational wave detector is already being designed and tested. LISA, Laser Interferometer Space Antenna, is a European Space Agency-led venture which will operate three spacecraft in a triangular formation, with the distance betwen each satellite being 2.5 million kilometers!
Just as multiple electromagnetic and radio telescopes around the world (and more in space!) complement each other's scientific missions, so too will the growing number and design of gravitational wave observatories.